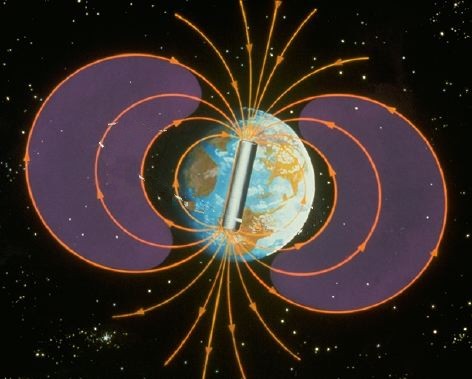
No other manifestation of Earth has
been as seemingly inexplicable as
the Earth’s magnetic field. Albert
Einstein is said to have considered
its origin one of the most important
unsolved problems in physics.
More than a thousand years ago,
individuals in China set afloat in
bowls of water tiny slivers of
loadstone, the mineral now called
magnetite, and discovered that the
slivers quickly assumed a preferred
direction. That observation led to
the development of the magnetic
compass.
In 1600, William Gilbert,
shown at right,
published
De Magnete, based
upon his extensive collection of
magnetic measurements from around
the globe, which showed that the
Earth itself is like a giant magnet,
rather than the magnetism arising
from an extraterrestrial source as
supposed by others [1].
In 1838, the
mathematical genius, Johann Carl
Friedrich Gauss, proved that the
Earth’s magnetism source is at, or
very near, the center of the Earth
[2].
The Earth acts like a giant magnet
with a magnetic field extending into
interplanetary space, shielding the
planet by deflecting charged
particles of the solar wind, as
illustrated at right, but it
is not a permanent magnet. Inside Earth is too hot (above
the Curie point) for a permanent
magnet to remain magnetized.
Moreover, the Earth’s magnetic field
must continuously be fed with
energy; otherwise its interactions
with the solar wind and with matter
in the Earth would cause it to decay
and eventually disappear. Thus,
there must exist at or near the
Earth’s center a mechanism for
generating the geomagnetic field and
an energy source for powering it.
In 1919, Larmor suggested that the
Sun’s magnetic field might be
sustained by a mechanism similar to
a self-exciting dynamo [3]. Elsasser
[4-6] and Bullard [7] first adapted
the solar dynamo concept to explain
the Earth’s magnetic field being
generated by a dynamo-like action in
the Earth’s fluid core.
The dynamo mechanism is essentially
a magnetic amplifier. Since 1939,
the geomagnetic field has been
believed to originate by convective
motions in the Earth’s fluid,
electrically-conducting core. The
idea is that the convective fluid
interacts with the Coriolis forces
produced by planetary rotation and
acts like a dynamo which is a
magnetic amplifier [8]. The idea
seemed so logical and seemed to make
such sense in explaining the origin
of the observed geomagnetic field
that for decades no one seriously
questioned whether long-term,
sustained convection could in fact
really occur in Earth’s fluid core.
Then along came J. Marvin Herndon
who, not only questioned it, but
discovered two reasons that
convection is physically impossible
in the Earth's fluid core
[9-11].
Too often scientists toss about the
term convection without stopping to
think what it actually means and
entails. Nobel Laureate Subramanyan
Chandrasekhar, pictured at right, defined convection in
the following way [12]:
“The
simplest example of thermally
induced convection arises when a
horizontal layer of fluid is heated
from below and an adverse
temperature gradient is maintained.
The adjective ‘adverse’ is used to
qualify the prevailing temperature
gradient, since, on account of
thermal expansion, the fluid at the
bottom becomes lighter than the
fluid at the top; and this is a
top-heavy arrangement which is
potentially unstable. Under these
circumstances the fluid will try to
redistribute itself to redress this
weakness in its arrangement. This is
how thermal convection originates:
It represents the efforts of the
fluid to restore to itself some
degree of stability.”
From studies of rock-magnetism, it
is known that the geomagnetic field
has existed for at least 3.5 billion
years. Except for reversals of
polarity, the geomagnetic field has
been remarkably stable for long
periods of time. In fact, at times
the geomagnetic field persisted for
at least forty million years without
reversals. So, if the geomagnetic
field is produced by a dynamo
mechanism, then long-term, stable
convection must necessarily prevail
in the operant fluid of the dynamo.
But, can stable convection exist for
extended periods of time within
Earth’s fluid core? This is the
question J. Marvin Herndon, pictured
at left, addressed
[9-11].
For stable convection to exist for
extended periods of time in the
Earth’s fluid core, it is necessary
that an adverse temperature gradient
be maintained for extended periods
of time so that heat produced
beneath the fluid core will cause
the fluid at the bottom of the core
to be lighter, more buoyant, making
it rise to the top of the core,
bringing the heat with it. To
maintain that “adverse temperature
gradient” for extended periods of
time requires the temperature at the
top of the fluid core to be
maintained at a lower temperature
than at the bottom of the fluid
core. The top of the fluid core can
only remain cooler than the bottom
of the core if the heat brought to
top by convection and by thermal
conduction can be efficiently
removed. And, therein lays one
problem.
The Earth’s fluid core is wrapped in
an insulating blanket, a rock shell, the mantle, that is 2900 km thick,
and which has a considerably lower heat capacity and lower thermal
conductivity than the fluid core. Thus heat brought to the top of the
core cannot be efficiently removed by thermal conduction. In other
words, in the fluid core, an adverse temperature gradient cannot be
maintained for extended periods of time, and thus convection cannot be
sustained for extended periods of time [9-10].
But, as Herndon discovered, there is
another, even more serious, reason that convection is physically
impossible in the Earth's fluid core. Because of the over-burden weight,
the Earth’s core is about 23% more dense at the bottom than at the top.
The tiny, tiny amount of thermal expansion at the bottom cannot make the
Earth’s core top-heavy and cannot cause a thermally-expanded “parcel”
from the bottom to float to the top of the core as required for
convection. Thus, the Earth’s core cannot engage in convection [11].
So, the implication is quite
clear: Either the geomagnetic field
is generated by a process other than
the convection-driven dynamo-mechanism, or there
exists another fluid region within
the deep-interior of Earth which can
sustain convection for extended
periods of time. The latter appears
to be the case.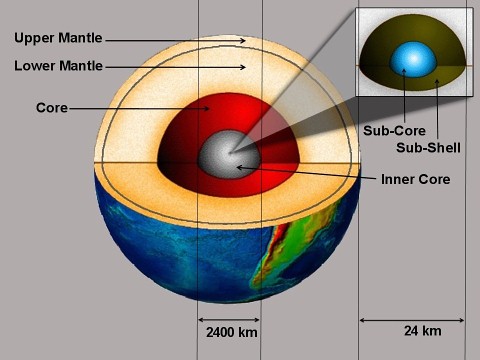
In 1993, J. Marvin Herndon published
the first of a series of scientific
articles revealing the background,
feasibility and evidence of a
nuclear fission reactor, called the
georeactor, at the center of the
Earth as the energy source for the
geomagnetic field [9-11,13-20], which, as Rao notes with extensive references
[21], may offer the solution to the
riddles of geomagnetic field
variability and deep-Earth helium
production.
The calculations
underlying Herndon’s georeactor
concept have been verified [22] and
extensive numerical simulations have
been conducted at Oak Ridge National
Laboratory [17, 18] and using
computer software licensed there
from [19, 20].
In 1996, in a
scientific article published in the
Proceedings of the National
Academy of Sciences USA, Herndon
described the georeactor as
consisting of a uranium sub-core
surrounded by a sub-shell consisting
of fission products and products of
radioactive decay which may be “a
slurry or a fluid” as illustrated at
right [15].
In
2007, Herndon presented evidence to support his idea of the georeactor
sub-shell being a slurry or fluid, and suggested that the Earth’s
magnetic field is produced by a dynamo-mechanism operating in the
georeactor sub-shell [9]. Significantly, within the georeactor sub-shell
there is no impediment to long-term, sustained convection; heat
generated by nuclear fission in the sub-core causes the fluid at the
bottom of the sub-shell to be lighter, more buoyant, making it rise to
the top of the sub-shell, where it contacts the relatively good thermal
conductor heat-sink that is the inner core, which is in contact with
another relatively good thermal conductor heat-sink, Earth’s fluid core.
Thus, there is no impediment to long-term, sustained convection in the
georeactor sub-shell [9-11].
It is expected that convective
motions within the electrically
conducting fluid (or slurry)
sub-shell will interact with the
Coriolis forces produced by
planetary rotation and act like a
dynamo, a magnetic amplifier, as
illustrated at right. And, unlike in Earth’s fluid
core, the georeactor sub-shell
contains large amounts of
neutron-rich radioactive
fission-produced elements which beta
decay yielding electrons for
generating magnetic seed-fields for
amplification. The georeactor unit
thus acts as both the energy source
and the operant fluid for generating
the Earth’s magnetic field by dynamo
action.
Even though variations in nuclear fuel
occur over time, Herndon's georeactor uniquely is expected to be
self-regulating through establishing a balance between heat-production
and actinide settling-out [11]. In the micro-gravity environment at the
center of Earth, georeactor hear production that is too energetic would
be expected to cause actinide sub-core disassembly, mixing actinide
elements with neutron-absorbers of the sub-shell, quenching the nuclear
fission chain reaction. But as the denser actinide elements begin to
settle-out of the mix, the chain reaction would re-start, ultimately
establishing a balance, an equilibrium between heat-production and
actinide settling-out, a self-regulating mechanism.
Because
of the self-regulation mechanism, the periods of long-term stable
magnetic field production lasting 40+ million years, the so-called "superchrons",
are expected. But, what then causes magnetic reversals?
The Earth's
magnetic field has been decreasing in intensity over the past hundred
years. Moreover, as shown in the figure at left, the North Magnetic Pole
has recently moved at a rapid rate toward Siberia. These observations
are taken by some as a possible indication of a forth-coming magnetic
reversal, potentially the first in some 700,000 years.
External influences, Herndon
suggests, intermittently disrupt the stability of georeactor geomagnetic
field generation and possibly lead to a magnetic reversal. For example,
electrical currents induced by superintense solar outbursts could cause heating in the georeactor
sub-shell, possibly disrupting convection. Severe Earth trauma might
also disrupt convection in the georeactor's sub-shell.
Because the georeactor mass is about one ten-millionth that of the fluid
core, such changes might occur far more quickly than previously assumed.
As described by Herndon
[11], georeactor sub-shell dimensions, mass, and inertia are orders of
magnitude less than those of the Earth’s core, meaning that changes in
the geomagnetic field, including reversals and excursions, can take
place on much shorter time-scales than previously thought. External
effects that potentially de-stabilize convection in the georeactor sub-shell
assume greater importance and pose the possibility of reversals
occurring on a time scale measured in months or years.
Currently
active internally generated magnetic fields have been detected in six
planets (Mercury, Earth, Jupiter, Saturn, Uranus, and Neptune) and in
one satellite (Jupiter’s moon Ganymede).
Magnetized surface areas of Mars and the Moon indicate the former
existence of internally generated magnetic fields in those bodies.
J. Marvin
Herndon has presented evidence attesting to the commonality of matter in
the Solar System, which is like that of the deep-interior of Earth, and
has made the suggestion [19, 22] that planetary magnetic fields
generally arise from the same georeactor-type mechanism which Herndon
[9-11] has suggested generates and powers the Earth’s magnetic field.
YouTube Video:
Origin of Earth's Magnetic Field
(click here) This video is
best "watched in high quality"
as it contains an experimental
demonstration of why long-term
convection, and hence
dynamo-action, in the fluid core
is impossible.
Return to Home Page
NuclearPlanet.com
|